Maltose or malt sugar or α-D-glucopyranosyl-(1→4)-D-glucopyranose is a disaccharide made up of two molecules of D-glucose in the pyranose form. The two monosaccharides are joined by an α-(1→4) glycosidic bond, a covalent bond between C1 of a glucose unit, its hemiacetal anomeric carbon, and the oxygen atom of hydroxyl group at C4 of the other glucose unit. The bond occurs with retention of the C1 configuration, namely, alpha. Since one glucose residue retains a hemiacetal carbon, maltose is a reducing sugar.[10]
It is produced in the proximal part of the small intestine, the duodenum, and in germinating seeds by the action of amylases on starch.[1][4] It is produced industrially by acidic or enzymatic hydrolysis, for example by fungal or bacterial amylases, such as those from Aspergillus oryzae or Bacillus subtilis, respectively, of starches of different origins.
It does not occur naturally in foods, and is found only in some processed foods in which it is added during processing. It is found, for example, in many bakery and pastry products, in which it acts as a sweetener, stabilizer, and preservative.[16] Therefore, it can be counted among the authorized food additives.[9]
In the duodenum, the α-(1→4) glycosidic bond is hydrolyzed in a reaction catalyzed by hydrolases of the brush border of enterocytes; the released glucose is absorbed and passes into the bloodstream.[14]
Contents
Chemical properties
As with the disaccharides lactose, sucrose, and trehalose, its molecular formula is C12H22O11 and molecular weight is 342.30 g/mol.[9]
It is very soluble in water and has a sweet taste, but is only about 33% as sweet as sucrose.
Like monosaccharides, and lactose among disaccharides, it is a reducing sugar, since the α-(1→4) glycosidic bond does not affect the hemiacetal (anomeric) carbon of one of the two glucose residues, which is then free to revert back to the carbonyl form, namely, in solution its ring can open to expose a free aldehyde group.[10] It should be emphasized, however, that the open-chain aldehyde form is present in very small amounts.
The anomeric carbon not involved in the glycosidic bond exhibits mutarotation, that is, both the α and β configurations are allowed, even if the β configuration is the predominant anomeric form.
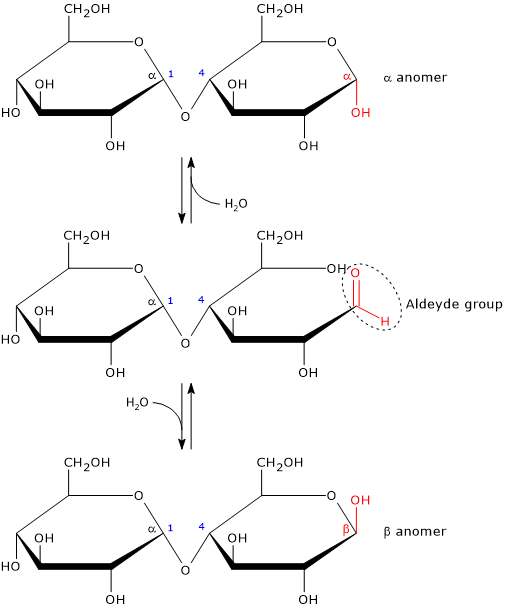
Anomerism is a type of optical isomerism characteristic of carbohydrates. Two stereoisomers of a cyclic monosaccharide are defined anomers if they differ only in the configuration of the hemiacetal or hemiketal carbon, which is called anomeric carbon or anomeric center.[15]
What happens is that the intramolecular cyclization of monosaccharides causes the carbon atom of the carbonyl group to become an asymmetric carbon, namely, a chirality center. And in solution, in equilibrium with their open form, two anomers can be formed:
- α-isomer, if, during cyclization, the oxygen of the hydroxyl group attacks the carbon atom of the carbonyl group from topside of the sp2 plane;
- β-isomer, if the oxygen of the hydroxyl group attacks the carbon atom of the carbonyl group from bottom side of the sp2 plane.
Role
During seed germination, endospermic starch is hydrolyzed by amylases to maltose and glucose, which concur to support, along with other hydrolysis products, the growth of the germinating seedlings.[1] Even the production of alcoholic beverages made by the fermentation of cereals, of foods with a high maltose content, such as glucose and maltose syrups, or bread making exploit amylase action on starch for the release of the disaccharide.[4]
In pastry making it is used both as a sweetener and stabilizer for icings, of which it does not increase the sweetness as sucrose would. Furthermore, being able to inhibit the retrogradation of starch, it can prolong its shelf life.[16] For these reasons, maltose can be classified as preservatives, stabilizers or sweeteners for food use.
It is present in many carbohydrate preparations for infant feeding.
Outside the food industry, it is used, for example, as a stabilizer for immunoglobulins.[9]
Maltose digestion
In humans, carbohydrate digestion begins in the oral cavity, with salivary alpha-amylase, and continues and ends in the duodenum, by the action of hydrolases such as pancreatic alpha-amylase and those of the brush border of enterocytes. The combined action of these enzymes allows hydrolysis of disaccharides, oligosaccharides and polysaccharides into the constituent monosaccharides: fructose, glucose, and galactose. The absorption of monosaccharides occurs in the small intestine, and is mediated by specific protein transporters present in the plasma membrane of enterocytes.[14]
The action of salivary and pancreatic alpha-amylase on the two polysaccharides that make up starch, amylose and amylopectin, leads to the release of maltose, maltotriose, an oligosaccharide made up of three glucose molecules joined by α-(1→4) glycosidic bonds, and, from amylopectin, also α-limit dextrins, glucose polymers with at least one α-(1→6) glycosidic bond.[2][3]
Although maltose, maltotriose and α-limit dextrins can be obtained from glycogen breakdown, such source has a negligible role since, after the death of the animal, glycogen undergoes rapid degradation, mostly to glucose and lactic acid.
The α-(1→4) glycosidic bond of maltose is hydrolyzed in a reaction that can be catalyzed by two enzymes: sucrase-isomaltase (EC 3.2.1.48 and 3.2.1.10) and maltase-glucoamylase or MAG (EC 3.2.1.20 and 3.2.1.3).[11]
Sucrase-isomaltase is a bifunctional enzyme that has two active sites.[7] One active site, the sucrase, is an alpha-glucosidase that hydrolyzes glycosidic bonds of maltose, sucrose, and short α-(1→4) linked glucose oligomers with up to six glucose units.[13] Sucrase is responsible of 60-80 percent of small bowel maltase activity.[5] The other active site, the isomaltase, is an α-(1→6) glycosidase that catalyzes the release of straight chains from α-limit dextrins.[12]
Like sucrase-isomaltase, maltase-glucoamylase is an enzyme with two active sites. One active site has a high specificity against maltose; the other has a broad substrate specificity and acts against glucose oligomers. Both active sites catalyze the release of glucose units.[11]
Note that alpha-amylase, sucrase-isomaltase and maltase-glucoamylase, working in synergy, completely digest dietary starches into glucose units.
Sucrase-isomaltase and MAG deficiency
The deficit of just one glycosidase of the brush border of enterocytes is generally due to a genetic defect, whereas the lack of all glycosidases is often the result of an intestinal infection. After the infection has been cured, these enzymes gradually recover.
Both sucrase and isomaltase activities can be affected in congenital or primary sucrase-isomaltase deficiency, with accumulation, in the first case also of indigested maltose. A similar situation can occur in the case of congenital maltase-glucoamylase deficiency, a rather rare condition with few cases described in the literature.[6] In both cases, as well as during intestinal infections, undigested carbohydrates remain in the intestinal lumen, where they can be partially fermented by gut microbiota, which is part of the human microbiota, leading to an excessive production of gas, such as hydrogen, carbon dioxide, and methane, and short-chain fatty acids, mainly acetic acid, propionic acid, and butyric acid.[8] The presence of indigested carbohydrates and the products of their fermentation, many of which are osmotically active solutes, causes an increase in intraluminal osmotic pressure, an influx of water into the lumen, and subsequent diarrhea.[17]
Treatment is to reduce or avoid dietary maltose.
References
- ^ a b Andriotis V.M., Saalbach G., Waugh R., Field R.A., Smith A.M. The maltase involved in starch metabolism in barley endosperm is encoded by a single gene. PLoS One 2016;11(3):e0151642. doi:10.1371/journal.pone.0151642
- ^ Benjamin S., Smitha R.B., Jisha V.N., Pradeep S., Sajith S., Sreedevi S., Priji P., Unni K.N., Sarath Josh M.K. A monograph on amylases from Bacillus spp. Adv biosci biotechnol 2013;4:No.2. doi:10.4236/abb.2013.42032
- ^ Butterworth P.J., Warren F.J. and Ellis P.R. Human α-amylase and starch digestion: an interesting marriage. Starch/Stärke 2011;63:395-405. doi:10.1002/star.201000150
- ^ a b Garrett R.H., Grisham C.M. Biochemistry. 4th Edition. Brooks/Cole, Cengage Learning, 2010
- ^ Gericke B., Schecker N., Amiri M., Naim H.Y. Structure-function analysis of human sucrase-isomaltase identifies key residues required for catalytic activity. J Biol Chem 2017;292(26):11070-11078. doi:10.1074/jbc.M117.791939
- ^ Gurram B. – 11- Diarrhea. Editor(s): Kliegman R.M., Lye P.S., Bordini B.J., Toth H., Basel D. Nelson Pediatric Symptom-Based Diagnosis. Elsevier, 2018. Pages 182-203.e1. doi:10.1016/B978-0-323-39956-2.00011-X
- ^ Hauri H.P., Quaroni A., Isselbacher K.J. Biogenesis of intestinal plasma membrane: posttranslational route and cleavage of sucrase-isomaltase. Proc Natl Acad Sci U S A 1979;76(10):5183-6. doi:10.1073/pnas.76.10.5183
- ^ Holtug K., Clausen M.R., Hove H., Christiansen J., Mortensen P.B. The colon in carbohydrate malabsorption: short-chain fatty acids, pH, and osmotic diarrhoea. Scand J Gastroenterol 1992;27(7):545-52. doi:10.3109/00365529209000118
- ^ a b c National Center for Biotechnology Information. PubChem Compound Summary for CID 6255, Maltose. https://pubchem.ncbi.nlm.nih.gov/compound/Maltose. Accessed Jan. 2, 2024
- ^ a b Nelson D.L., Cox M.M. Lehninger. Principles of biochemistry. 6th Edition. W.H. Freeman and Company, 2012
- ^ a b Nichols B.L., Eldering J., Avery S., Hahn D., Quaroni A., Sterchi E. Human small intestinal maltase-glucoamylase cDNA cloning. Homology to sucrase-isomaltase. J Biol Chem 1998;273(5):3076-81. doi:10.1074/jbc.273.5.3076
- ^ Quezada-Calvillo R., Robayo-Torres C.C., Ao Z., Hamaker B.R., Quaroni A., Brayer G.D., Sterchi E.E., Baker S.S., Nichols B.L. Luminal substrate “brake” on mucosal maltase-glucoamylase activity regulates total rate of starch digestion to glucose. J Pediatr Gastroenterol Nutr. 2007 Jul;45(1):32-43. doi:10.1097/MPG.0b013e31804216fc
- ^ Quezada-Calvillo R., Sim L., Ao Z., Hamaker B.R., Quaroni A., Brayer G.D., Sterchi E.E., Robayo-Torres C.C., Rose D.R., Nichols B.L. Luminal starch substrate “brake” on maltase-glucoamylase activity is located within the glucoamylase subunit. J Nutr 2008:138(4);685-92. doi:10.1093/jn/138.4.685
- ^ a b Rosenthal M.D., Glew R.H. Medical biochemistry: human metabolism in health and disease. A John Wiley & sons, Inc., Publication, 2009
- ^ Soderberg T. Organic chemistry with a biological emphasis. Volume II. Chemistry Publications. 2019 https://digitalcommons.morris.umn.edu/chem_facpubs/2
- ^ a b Wang S., Li C., Copeland L., Niu Q., Wang S. Starch Retrogradation: a comprehensive review. Compr Rev Food Sci Food Saf 2015;14:568-585. doi:10.1111/1541-4337.12143
- ^ Weijers H.A., va de Kamer J.H., Mossel D.A., Dicke W.K. Diarrhoea caused by deficiency of sugar-splitting enzymes. Lancet. 1960;2(7145):296-7. doi:10.1016/s0140-6736(60)91381-7