Lactose or milk sugar or beta-D-galactopyranosyl-(1 → 4)-D-glucose is a disaccharide made up of one β-D-galactose molecule and one D-glucose molecule, in pyranose form. The two monosaccharides are joined by a β-(1→4) glycosidic bond. Since glucose has a hemiacetal carbon, lactose is a reducing sugar and has two anomeric forms, referred to as alpha and beta.[12]
It is synthesized by epithelial cells of the mammary gland during lactation through a condensation reaction between UDP-galactose and glucose.[1] The reaction is catalyzed by lactose synthase (EC 2.4.1.22), an heterodimeric complex made up of alpha-lactalbumin and beta-1,4-galactosyl transferase 1 (EC 2.4.1.38).[9]
In adults it accounts for 5-10 percent of dietary carbohydrates and, together with starch and sucrose, is one of the three most common dietary carbohydrates.[11]
In the duodenum and jejunum, the β-(1→4) glycosidic bond of lactose is hydrolyzed to yield glucose and galactose. The reaction is catalyzed by lactase (EC 3.2.1.108), a hydrolase of the brush border membrane of the enterocytes.[17]
Hypolactasia or lactase deficiency refers to the condition in which there is an insufficient lactase activity to digest lactose. It causes lactose malabsorption, and may be accompanied by lactose intolerance, which has only been recognized in the last 50 years and is the most common food intolerance.[5]
Lactose performs many functions, such as providing about 40 percent of the energy that the infant gets from breast milk and being a source of galactose, which is essential in the biosynthesis of glycosylated macromolecules required for the proper development of the nervous system.[3]
It is present in milk-derived products, in amounts inversely related to the degree of ripening.[4]
It is one of additives used in food and pharmaceutical industries.[15][16]
Contents
Chemical properties
As with the disaccharides sucrose, maltose and trehalose, its molecular formula is C12H22O11 and molecular weight is 342.30 g/mol.[12]
It is less soluble than the other disaccharides, and is about 16 percent as sweet as sucrose.
The glycosidic bond between galactose and glucose has β configuration, that is, the bond from the anomeric carbon of galactose is directed upwards. The β-(1→4) glycosidic bond is also present in chitin and cellulose.[16]
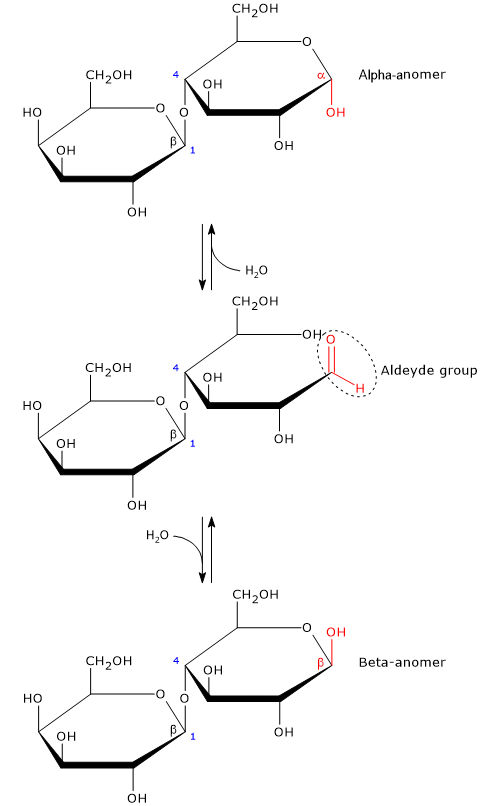
Like monosaccharides, and maltose among disaccharides, it is a reducing sugar, since the β-(1→4) glycosidic bond does not affect the hemiacetal (anomeric) carbon of the glucose molecule; therefore, in solution glucose ring can open and expose an aldehyde group.[13]
The open-chain or acyclic form of glucose is thermodynamically unstable, and, in solution, it is present only in trace amounts, less than 0.02 percent. The ring-closing reaction can yield two cyclic pyranose configuration, differing in the configuration of the hemiacetal carbon. This phenomenon is called mutarotation, and the resulting isomers are called alpha-anomer and beta-anomer. In solution, at 20 °C the beta configuration is the predominant anomeric form, about 63 percent, although the equilibrium ratio changes as a function of temperature, and only the beta anomer is present at temperatures >93.5 °C.[8]
Anomerism is a type of optical isomerism characteristic of carbohydrates, in which the cyclic monosaccharide differs only in the configuration of the hemiacetal or hemiketal carbon, known as the anomeric carbon or anomeric center.
Biosynthesis
Lactose is synthesized by epithelial cells of the mammary gland during lactation, in the reaction catalyzed by the lactose synthase system, a heterodimer present in the Golgi apparatus and made up by beta-1,4-galactosyl transferase 1 (EC 2.4.1.38), an intrinsic protein of the Golgi apparatus membrane, and alpha-lactalbumin, in a 1:1 ratio.[9] This protein-protein interaction is reversible and is promoted by monosaccharides and UDP-galactose.[24]
Lactose synthase catalyzes the reaction between UDP-galactose and glucose, that is, the transfer of galactose to glucose to form a β-(1→4) glycosidic bond between the C1 of galactose and the oxygen atom of the 4-hydroxyl group of glucose.[1]
Glucose is the primary precursor of monosaccharides that make up lactose: all of the glucose and about 70 percent of galactose, respectively.
The formation of the complex between beta-1,4-galactosyl transferase 1 and alpha-lactalbumin occurs in the lumen of the Golgi apparatus. Alpha-lactalbumin, a secretory protein, during its passage through the cell is present as soluble and transient protein in the lumen of the Golgi apparatus, where it binds, reversibly, to beta-1, 4-galactosyl transferase 1. The transient nature of the interaction between alpha-lactalbumin and the galactosyl transferase ensures a linkage between milk protein synthesis and lactose synthesis during lactation.[24]
Lactose cannot cross the membrane of the Golgi apparatus, and, with alpha-lactalbumin, is packaged into secretory vacuoles, whose contents is released from the cell by exocytosis.[2]
Alpha-lactalbumin
Lactalbumin is one of the main whey proteins and is catalytically inactive.
The synthesis of lactose requires the presence of alpha-lactalbumin, whose gene transcription is regulated by prolactin, also called luteotropin or luteotropic hormone. What happens is that, after childbirth, the reduction of progesterone levels leads to an increase in the synthesis of prolactin which stimulates the synthesis of alpha-lactalbumin in the epithelial cells of the mammary gland, and consequently the formation of lactose synthase system. Conversely, in non-lactating mammary gland, alpha-lactalbumin is not produced, lactose synthase system is not formed and lactose is not synthesized.[14]
Beta-1,4-galactosyl transferase 1
In mammals, beta-1,4-galactosyl transferase 1 is part of a group of seven galactosyl transferases which catalyze the formation of β-(1→4) glycosidic bonds between galactose and different acceptors.[24] It is expressed in most tissues where it catalyzes the addition of galactose to the 4-hydroxyl group of a non-reducing terminal N-acetyl-beta-D-glucosamine residue of glycoproteins and glycolipids.[14]
Beta-1,4-galactosyl transferase 1 has a low affinity for glucose, with a Km of about 2 mol/L.[2] During pregnancy, the enzyme is produced and stored in the epithelial cells of the mammary gland. The bond with alpha-lactalbumin triggers conformational changes in the active site region that increase its affinity for glucose by about 1000 times, and therefore decrease the Km for the monosaccharide to about 2 mmol/L. This allows, at physiological glucose concentrations, the preferential and efficient synthesis of lactose rather than the galactosylation of other acceptors.[24]
Food sources
Lactose is present in the milk of almost all mammalian species, although in different amounts among species. For example, it accounts approximately 7.5 percent and 4.5 percent of human and cow milk composition, respectively.[6] Its concentration during lactation, on the other hand, remains relatively stable.[7]
It is absent in the milk of a few species of mammals, like several species of the Otariidae family, such as the Cape fur seal (Arctocephalus pusillus pusillus) or the California sea lion (Zalophus californianus), or of the Phocidae family, such as the hooded seal (Cystophora cristata). In the Cape fur seal, the ability to produce lactose was lost due to mutations in the alpha-lactalbumin gene causing the absence of the protein.[19]
In dairy products, lactose is present in variable amounts, inversely related to the degree of ripening. Products such as yogurt or ricotta have the largest amounts, whereas hard cheeses, such as Parmigiano Reggiano, have the lowest amounts, often close to zero.[4]
It is present in very high amounts in products such as powdered milk and powdered whey.[15]
Food sources (100 g) | Lactose (g) |
Human milk | 7.5 |
Whole cow’s milk | 4.8 |
Semi skimmed cow’s milk | 4.9 |
Skimmed cow’s milk | 4.9 |
Powdered milk | 35.1 |
Skim milk powder | 50.5 |
Whey powder | 58-62 |
Buffalo milk | 4.9 |
Goat milk | 4.2 |
Yogurt | 3-4 |
Cow’s milk ricotta | 4.0 |
Fresh cheeses such as mozzarella | 1-3 |
Emmenthal and semi-hard cheeses | 0.1 |
Parmigiano Reggiano, Grana Padano and hard cheeses | 0 |
It is present in many processed foods such as candies, chocolate, breakfast cereals, baked goods, and cured meats, in which it is used as a food additive.[4]
It is also used in the pharmaceutical industry, in concentrations of the order of milligrams, in the production of tablets and inhalers.[15]
Lactose digestion
In humans, and in mammals in general, carbohydrate digestion occurs mainly in the duodenum and jejunum. Pancreatic alpha-amylase and the disaccharidases of the brush border of enterocytes hydrolyze polysaccharides, oligosaccharides and disaccharides into the constituent monosaccharides, namely, glucose, galactose and fructose. In the last step, absorption of monosaccharides occurs.[17]
The β-(1→4) glycosidic bond of lactose is hydrolyzed by lactase or lactase-phlorizin hydrolase. Under physiological condition, lactase is present at birth as it is crucial for the digestion of lactose.[23] The enzyme has two active sites:
- one capable of hydrolyzing the β-(1→4) glycosidic bond, therefore a beta-glucosidase;
- the other with phlorizin hydrolase activity, capable of hydrolyzing phlorizin and glycolipids, such as ceramides to yield fatty acids and sphingosine.
Lactase activity is abundantly present in the mid-jejunum, and, among the hydrolases of the brush border of enterocytes, it is the only one capable of hydrolyzing the β-(1→4) glycosidic bond of lactose.[21]
Among disaccharidases, it is the last to appear during fetal life, about 8 weeks after conception. At 34 weeks’ gestation lactase activity is about one-third of that detected in full-term newborns, and reaches three quarters of that of full-term newborns between the 35 and 38 weeks’ gestation. In nearly all full-term newborns, lactase synthesis and activity are high and remain so throughout the first 4 years of life.[20]
Among the hydrolases of the brush border, lactase is often the first to be lost during intestinal diseases as it is expressed late in the development of enterocytes, when they have almost reached the tip of the villus, and protrudes from the membrane.[20]
Role
Lactose plays anabolic and catabolic roles.
It acts as energy source, providing about 40 percent of energy babies get from mother’s milk.[18] In fact, the released glucose may enter glycolysis to produce ATP. However, depending on the cell’s need, glucose may be used for anabolic purposes, such as fatty acid synthesis, and then for the synthesis of triglycerides. On the other hand, galactose has a predominantly anabolic role. Once it has reached the liver, it enters the Leloir pathway and, converted into UDP-glucose, is used for glycogen synthesis.[3] Although in theory glucose 1-phosphate produced in the Leloir pathway may enter the glycolytic pathway, after conversion to glucose-6-phosphate, it seems that only a small percentage follow this pathway.
In the newborn and early childhood period, galactose is used in the biosynthesis of glycosylated macromolecules such as mucoproteins, cerebrosides, and gangliosides, which are components of the myelin sheaths of nerve cells. When myelination of nerve fibers and development are ended, the requirement for galactose is also greatly reduced; perhaps this may explain, from a physiological point of view, the decreased or absent ability of many populations to digest lactose already at a young age.[3]
Some antigens present on the membrane of red blood cells, in particular those used to determine the ABO group, have galactose residues.
Lactose is the main osmotic constituent in milk. As it can’t cross the membrane of the Golgi apparatus, it acts as an osmolyte and aids in drawing water. Such water flux into these organelles, and therefore into the mammary epithelial cells, is important in determining the volume of the milk.[24]
The bond between the two monosaccharides to make up lactose allows to reduce the osmotic pressure of the milk, which is equal to that of maternal plasma.[16]
It plays a role in intestinal absorption of minerals, especially during childhood. In fact, glucose and galactose are mostly absorbed by SGLT1 which cotransports sodium, calcium and water.[22]
The souring of milk is due to lactose fermentation to lactic acid by bacteria.
When undigested lactose reaches the colon, it can be metabolized by the gut microbiota, which is part of the human microbiota, and so it can act as a prebiotic.[11]
Hypolactasia
Hypolactasia or lactase deficiency, which may have a genetic basis or be secondary to damage to the enterocytes, causes lactose malabsorption which, once it reaches the colon, may give rise to gastrointestinal symptoms, a condition known as lactose intolerance.[5]
In the colon, the disaccharide can be partially fermented by the bacteria of the gut microbiota with production of an excess of gas, such as molecular nitrogen and hydrogen, carbon dioxide, methane, and short-chain fatty acids, mainly acetic acid, propionic acid and butyric acid. In addition, some of the fermentation products and the undigested and unfermented carbohydrates, osmotically active solutes, causes an increase in intraluminal osmotic pressure which leads to an increase in the water content of the colon.[11]
The symptoms that characterize lactose intolerance, resulting from the processes described above, are:
- abdominal pain, due to the acidification of the colon contents and to an irritating effect on the mucosa resulting from excess short-chain fatty acids;
- flatulence, bloating and abdominal distension, caused by excessive gas production;
- diarrhea, as a result of the increased volume of water in the colon due to the presence of osmotically active solutes and to the reduction in the efficiency of water absorption due to the increased motor activity of the colon induced by excess short-chain fatty acids.[11]
It should be noted that the cascade of events described above is applicable to any sugar that is not absorbed in the small intestine, as well as too much fiber in the diet.
Hypolactasia is not always accompanied by lactose intolerance, the latter manifesting itself in 30-50 percent of hypolactasia subjects.[4] Why? The amount of residual lactase activity is undoubtedly fundamental. However, other reasons may be important too, and are briefly described below.
- The amount of lactose ingested, as the risk increases as the amount of the disaccharide increases.
- The stomach emptying time, which is influenced by the composition of the meal. For example, lipids, proteins, and fibers can effectively slowing down the emptying, allowing the disaccharide to enter the small intestine slowly.
- The intestinal transit time, which influences the contact time between the enzyme and lactose. Therefore, foods that slow down transit time may increase the probability of contact.
- The composition of colonic microflora, which may affect fermentation processes.
Primary hypolactasia
In approximately three quarters of the world’s population, as in most mammals, a reduction in the biosynthesis of lactase occurs after weaning. This reduction isn’t due to a damage to the gene that codes for this protein, present on chromosome 2, but is genetically programmed and transmitted by a recessive gene. The residual lactase activity can be equal to 5-10 percent of that present during infancy.[5]
For example, in Mediterranean populations, a decrease of lactase activity may occur as early as in infancy, up to of 90 percent than that present at birth. Among White North American the deficiency occurs in 5-20 percent of population, among Black North American it reaches to 70-75 percent, among Asians, Filipinos it reachs to 95 percent and Thai, the main ethnic group of Thailand, 99 percent as in Nigeria, Benin e Togo the Yoruba. Conversely, the deficiency occurs in only 3 percent of Danish and some nomadic, milk-consuming populations living in the arid zone of Arabia and North Africa.[5]
Therefore, the decline in lactase activity, called primary hypolactasia, is not a disease, should be considered phylogenetically normal, and is not affected by continuous exposure to milk or lactose.[20]
In primary hypolactasia, there isn’t an uniform distribution in the reduction of lactase activity, but rather a patchy distribution, even in the same villus, between enterocytes that do not produce lactase, which are present in higher number, and enterocytes produce high amounts.[10]
It seems that the persistence of lactase activity represented a survival advantage. With the spread of dairy farming, about 10,000 years ago, dairy consumption would have provided subjects with sufficient lactase activity an advantage for survival during severe winters in Northern Europe and in times of poor harvest.[4]
Secondary hypolactasia
Secondary hypolactasia is due to conditions causing damage to enterocytes, such as intestinal infections, cow’s milk protein allergy, Crohn’s disease, celiac disease, i.e. an autoimmune reaction to dietary gluten, drug therapies, surgery, radiation to the gastrointestinal tract, or excessive alcohol consumption. In these circumstances, many brush border proteins are affected and, among the glycosidases, lactase undergoes the greatest reduction.[5]
Secondary hypolactasia is most common in Third World nations where chronic gastrointestinal infections are prevalent.
Once the trigger has been resolved, the epithelium heals, lactase activity increases and eventually returns to normal. Complete resolution can take up to six months, during which time it is advisable to avoid milk and lactose-containing foods.[20]
Congenital lactose intolerance
Congenital lactose intolerance, a disease inherited in an autosomal recessive pattern, is an extremely rare inborn error of metabolism.[5] Lactase activity is undetectable, and affected newborn has severe diarrheal illness within the first days of life. The only treatment is a lactose-free diet that eliminates symptoms and allows normal growth and development.[20]
References
- ^ a b Baynes J.W., Dominiczak MH. Medical biochemistry. 5th Edition. Elsevier, 2019
- ^ a b Brew K. Milk proteins | α-Lactalbumin. Editor(s): Fuquay J.W. Encyclopedia of dairy sciences. 2nd Edition. Academic Press, 2011;780-786. doi:10.1016/B978-0-12-374407-4.00432-5
- ^ a b c Conte F., van Buuringen N., Voermans N.C., Lefeber D.J. Galactose in human metabolism, glycosylation and congenital metabolic diseases: time for a closer look. Biochim Biophys Acta Gen Subj 2021;1865(8):129898. doi:10.1016/j.bbagen.2021.129898
- ^ a b c d e Di Stefano M. Il malassorbimento e l’intolleranza al lattosio. Fisiopatologia, diagnosi e approccio terapeutico. SIMG 2012;5:40-45
- ^ a b c d e f Fassio F., Facioni M.S., Guagnini F. Lactose maldigestion, malabsorption, and intolerance: a comprehensive review with a focus on current management and future perspectives. Nutrients 2018;10(11):1599. doi:10.3390/nu10111599
- ^ Hennet T., Borsig L. Breastfed at Tiffany’s. Trends Biochem Sci 2016;41(6):508-518. doi: 10.1016/j.tibs.2016.02.008
- ^ Hovey R.C. The marvels of milk and lactation. Editor(s): Skinner M.K. Encyclopedia of reproduction. 2nd Edition. Academic Press, 2018;2:793-797. doi:10.1016/B978-0-12-801238-3.64696-2
- ^ Jawad R., Drake A.F., Elleman C., Martin G.P., Warren F.J., Perston B.B., Ellis P.R., Hassoun M.A., Royall P.G. Stability of sugar solutions: a novel study of the epimerization kinetics of lactose in water. Mol Pharm 2014;11(7):2224-38. doi:10.1021/mp400509t
- ^ a b Lin Y., Sun X., Hou X., Qu B., Gao X., Li Q. Effects of glucose on lactose synthesis in mammary epithelial cells from dairy cow. BMC Vet Res 2016;12:81. doi:10.1186/s12917-016-0704-x
- ^ Majuri L., Raja V., Potter J., Swallow D., Wan Ho M., Fiocca R., Finzi G., Cornaggia M., Capella C., Quaroni A. & Auricchio A. Mosaic pattern of lactase expression by villus enterocytes in human adult-type hypolactasia. Pediatr Res 1990;27:532. doi:10.1203/00006450-199005000-00040
- ^ a b c d Misselwitz B., Butter M., Verbeke K., Fox M.R. Update on lactose malabsorption and intolerance: pathogenesis, diagnosis and clinical management. Gut. 2019;68(11):2080-2091. doi:10.1136/gutjnl-2019-318404
- ^ a b National Center for Biotechnology Information. PubChem Compound Summary for CID 6134, beta-Lactose. https://pubchem.ncbi.nlm.nih.gov/compound/beta-Lactose. Accessed Dec. 12, 2023
- ^ Nelson D.L., Cox M.M. Lehninger. Principles of biochemistry. 6th Edition. W.H. Freeman and Company, 2012
- ^ a b Palmer T., Bonner P.L.. Monomeric and oligomeric enzymes. Editor(s): Palmer T., Bonner P.L. Enzymes (2nd Edition). Woodhead Publishing. 2011;76-83. doi:10.1533/9780857099921.1.76
- ^ a b c Portnoy M., and Barbano D.M. Lactose: use, measurement, and expression of results. J Dairy Sci 2021;104(7):8314-8325. doi:10.3168/jds.2020-18706
- ^ a b c Romero-Velarde E., Delgado-Franco D., García-Gutiérrez M., Gurrola-Díaz C., Larrosa-Haro A., Montijo-Barrios E., Muskiet F., Vargas-Guerrero B., & Geurts J. The Importance of lactose in the human diet: outcomes of a mexican consensus meeting. Nutrients 2019;11(11):2737. doi:10.3390/nu11112737
- ^ a b Rosenthal M.D., Glew R.H. Medical biochemistry: human metabolism in health and disease. A John Wiley & sons, Inc., Publication, 2009
- ^ Schaafsma G. Lactose and lactose derivatives as bioactive ingredients in human nutrition. Int Dairy J 2008;18:458-465. doi:10.1016/j.idairyj.2007.11.013
- ^ Sharp J.A., Lefèvre C., Nicholas K.R. Lack of functional alpha-lactalbumin prevents involution in Cape fur seals and identifies the protein as an apoptotic milk factor in mammary gland involution. BMC Biol 2008;6:48. doi:10.1186/1741-7007-6-48
- ^ a b c d e Suarez F., Shannon C., Hertzler S., Savaiano D. Food intolerance | Lactose Intolerance. Editor(s): Caballero B. Encyclopedia of food sciences and nutrition. 2nd Edition. Academic Press, 2003;2634-2642. doi:10.1016/B0-12-227055-X/00511-3
- ^ Troelsen J.T. Adult-type hypolactasia and regulation of lactase expression. Biochim Biophys Acta. 2005 May 25;1723(1-3):19-32. doi:10.1016/j.bbagen.2005.02.003
- ^ Wright E.M., Hirayama B.A., Loo D.F. Active sugar transport in health and disease. J Intern Med 2007;261(1):32-43. doi: 10.1111/j.1365-2796.2006.01746.x. PMID: 17222166
- ^ Zecca L., Mesonero J.E., Stutz A., Poirée J.C., Giudicelli J., Cursio R., Gloor S.M., Semenza G. Intestinal lactase-phlorizin hydrolase (LPH): the two catalytic sites; the role of the pancreas in pro-LPH maturation. FEBS Lett 1998;435(2-3):225-8. doi: 10.1016/s0014-5793(98)01076-x
- ^ a b c d e Zhang Y., Brew K. Milk proteins | Alpha-Lactalbumin. Editor(s): Roginski H. Encyclopedia of dairy sciences. Elsevier, 2002;1924-1932. doi:10.1016/B0-12-227235-8/00319-9