The Leloir pathway is the main pathway for galactose metabolism.
Discovered by Leloir L.F. and colleagues in 1948, it leads to the conversion of galactose into glucose 1-phosphate, namely, to the inversion of the configuration of hydroxyl group at C4 of galactose, one of the chirality centers of the monosaccharide.[9]
The metabolic intermediates involved in this isomerization are building blocks in many metabolic pathways, such as glycosylation reactions of proteins and lipids or glycogen synthesis, depending on the stage of development, the metabolic state of the cell, and the type of tissue.[6]
Except for the first step, the other reactions of the Leloir pathway may proceed in both directions, depending on substrate levels and metabolic demands of the tissue. This allows the interconversion of galactose and glucose.[4]
The importance of the Leloir pathway, and therefore of galactose, is emphasized by the fact that it is highly conserved in nature, from bacteria to plants and animals, and, in humans, by the severity of the consequences due to mutations in one of the genes encoding the enzymes of the pathway, mutations that cause the genetic metabolic disorder known as galactosemia.[2][8]
Contents
- Galactose
- The steps of the Leloir pathway
- What is the role of the Leloir pathway?
- Leloir pathway and galactosemia
- References
Galactose
Galactose, together with glucose and fructose, is one of the monosaccharides that can be absorbed in the intestine. The main dietary source of galactose is lactose, which, with maltose, trehalose and sucrose, is one of the disaccharides found in food. Since there are no transporters for disaccharides, in the last stage of carbohydrate digestion their glycosidic bonds are hydrolyzed with the release of the constituent monosaccharides, which for lactose are glucose and galactose. Then follows the absorption of monosaccharides which, through the portal system, reach the liver, which is the main site for the metabolism of galactose and absorbs, through passive diffusion mediated by the GLUT2 transporter, most of it, about 88%.[3] The residual circulating quantity reaches other organs and tissues, such as the mammary gland that, during the lactation phase, uses it for the production of lactose and the glycosylation of milk proteins and lipids.[4]
The steps of the Leloir pathway
The Leloir pathway consists of four reactions catalyzed by the enzymes galactose mutarotase or aldose 1-epimerase (EC 5.1.3.3), galactokinase (EC 2.7.1.6), galactose 1-phosphate uridylyltransferase or GALT (EC 2.7.7.12) and UDP-galactose 4-epimerase or GALE (EC 5.1.3.2).[8]
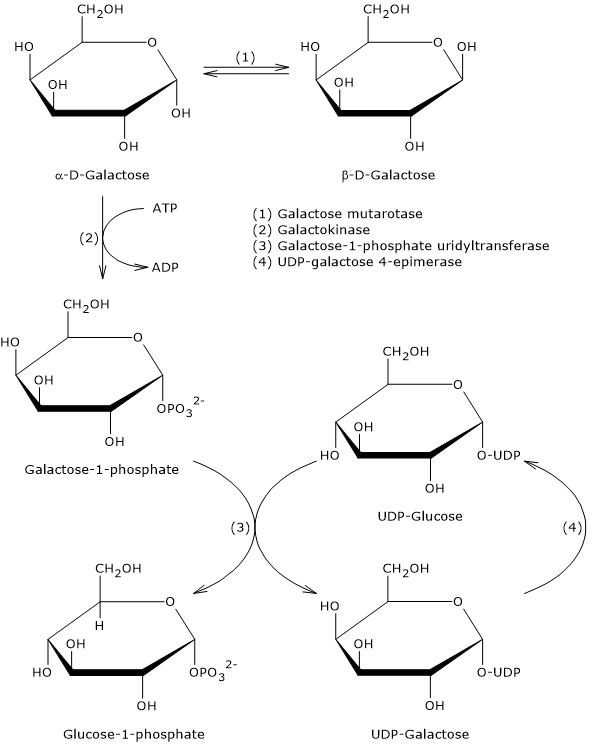
Step 1: galactose mutarotase
The cleavage of the β-(1→4) glycosidic bond of lactose leads to the release of glucose and beta-galactose.
Galactokinase, the enzyme that catalyzes the second step of the Leloir pathway, is specific for the alpha anomer of galactose. The conversion of beta-galactose to alpha-anomer is catalyzed by galactose mutarotase. The enzyme is also able to interconvert alpha and beta-configurations of glucose, xylulose, maltose and lactose, albeit with variable efficiency.[12]
Step 2: galactokinase
In the second step, alpha-galactose is phosphorylated to galactose 1-phosphate, in a reaction catalyzed by galactokinase.[8] The phosphorylation of galactose has some functions.
- Galactose 1-phosphate cannot diffuse out of the cell, as it is negative charged and there are no transporters for phosphorylated sugars in the plasma membrane.
- Phosphorylation increases the energy content of the monosaccharide, and this starts to destabilize the molecule facilitating its further metabolism.
- The phosphorylation of galactose maintains a low intracellular concentration of the monosaccharide, thus favoring its facilitated transport into the cell.
The reaction catalyzed by galactokinase is the irreversible step of the Leloir pathway.[4] Unlike hexokinase and glucokinase (EC 2.7.1.1), which phosphorylate the hydroxyl group at C6 of glucose, galactokinase and fructokinase (EC 2.7.1.4) phosphorylate the hydroxyl group at C1 of galactose and fructose, respectively.[11]
The conversion of galactose 1-phosphate to glucose 1-phosphate requires two reactions, the third and fourth of the Leloir pathway, respectively.
Step 3: galactose 1-phosphate uridylyltransferase
In the third step, galactose 1-phosphate uridylyltransferase catalyzes the transfer to galactose 1-phosphate of the UMP group of UDP-glucose, to form UDP-galactose and glucose 1-phosphate. The reaction proceeds with a ping-pong mechanism with the formation of a covalent intermediate between the enzyme and UMP.[7]
Step 4: UDP-galactose 4-epimerase
In the last step, UDP-galactose is converted to UDP-glucose in a reaction catalyzed by UDP-galactose 4-epimerase. The enzyme inverts the configuration of the hydroxyl group at C4, and is responsible for the interconversion of UDP-galactose and UDP-glucose, hence between galactose and glucose.[8]
UDP-galactose 4-epimerase requires NAD+ as a cofactor and the reaction proceeds through the formation of a ketonic intermediate at C4 with simultaneous reduction of NAD+ to NADH. Subsequently, the ketonic intermediate rotates presenting the opposite face of the sugar to NADH, and a hydride ion is transferred back from NADH to C4 but in the opposite configuration.[13] Since NAD is first reduced and then oxidized, no net oxidation or reduction of the coenzyme occurs, which therefore does not appear in the reaction equation. In mammals, UDP-galactose 4-epimerase also catalyzes the interconversion between UDP-N-acetylgalactosamine and UDP-N-acetylglucosamine.[3] It seems that UDP-galactose 4-epimerase is the rate-limiting enzyme of the Leloir pathway.
UDP-glucose produced is recycled in the reaction catalyzed by UDP-glucose pyrophosphorylase, with the release of glucose 1-phosphate.[6]
Note that UDP-galactose and UDP-glucose, or more generally galactose and glucose, have the same molecular formula. Therefore, they are isomers, and differ only in the configuration of the fourth carbon atom. Therefore they are an example of optical isomerism.
What is the role of the Leloir pathway?
The Leloir pathway allows the cell to use galactose or the derived glucose in many metabolic pathways, both anabolic and catabolic, depending on the metabolic state of the cell or tissue. Furthermore, since the reaction catalyzed by UDP-galactose 4-epimerase is reversible, conversion of glucose to galactose and nucleotide derivatives is possible.[4][6]
UDP-galactose can be used:
- in the glycosylation of proteins and lipids, such as galactocerebrosides which are the major glycolipids components in myelin, and for this reason galactose was initially called cerebrose;
- in the lactating mammary gland for the production of lactose in a reaction catalyzed by the lactose synthase system. Moreover, as the reaction catalyzed by UDP-galactose 4-epimerase may proceed in both directions, glucose can be converted, after activation to UDP-glucose in a reaction catalyzed by UDP-glucose pyrophosphorylase (EC 2.7.7.9), into UDP-galactose for the synthesis of lactose.
In the liver and skeletal muscle, UDP-glucose derived from UDP-galactose, can be used:[4]
-
- in the glycosylation of lipids and proteins;
- in glycogen synthesis, when the energy demand of the cell is low; and, with respect to glucose and fructose, galactose is preferentially incorporated in hepatic glycogen rather than being directed to oxidative metabolism;
- following conversion to glucose 1-phosphate, in a reaction catalyzed by UDP-glucose pyrophosphorylase, and isomerization to glucose 6-phosphate in a reaction catalyzed by phosphoglucomutase (EC 5.4.2.2), it may enter different metabolic pathways, such as glycolysis, the pentose phosphate pathway or gluconeogenesis.[3]
Note: UDP-galactose, discovered during the studies on the Leloir pathway, was the first nucleotide sugar to be identified.[1]
Leloir pathway and galactosemia
Glycosylations are post-translational modifications that play a key role in enabling and regulating many biological processes. Glycosylation defects have been related to many pathological conditions such as cancer, diabetes, and congenital inborn errors of metabolism such as congenital disorders of glycosylation, which are mainly autosomal recessive monogenic disorders.[5] Congenital disorders of glycosylation include galactosemia, which was first described by von Reuss A. in 1908.[14] Galactosemia is due to mutations in one of the genes encoding the four enzymes of the Leloir pathway, and four types have been identified:
- type I, due to deficiency of galactose 1-phosphate uridylyltransferase, the most common form, also called classical galactosemia;
- type II, due to galactokinase deficiency;
- type III, due to galactose 4-epimerase deficiency;
- type IV, due to galactose mutarotase deficiency.[15]
To date, the therapeutic standard of care for galactosemia is a galactose-restricted diet.
Galactosemia and cataracts
The accumulation of galactose fuels alternative metabolic pathways such as the synthesis of galactitol and galactonate.
The symptoms of galactosemia include the early onset of cataracts, usually within the first two years of life, and in the most severe cases, brain, liver, and kidneys damages, too.[12]
It seems that one of the factors triggering cataracts is the reduction of galactose, accumulated in the lens of the eye, to galactitol, in a reaction catalyzed by aldose reductase (EC 1.1.1.21).[10] Galactitol, which is poorly metabolized, does not diffuse through cell membranes due to its poor lipophilicity and, being osmotically active, increases the intracellular osmotic pressure causing a net flow of water inside the cell. Moreover, its synthesis, depleting NADPH levels, may reduce glutathione reductase (EC 1.8.1.7) activity and lead to free radical accumulation.[3]
The osmotic effect and the build-up of free radicals may ultimately damage cell integrity and cause cell death. Furthermore, it has been reported that galactitol is a galactose mutarotase inhibitor, so its accumulation may lead to further accumulation of unmetabolized galactose.[12]
References
- ^ Cardini C.E., Paladini A.C., Caputto R., Leloir L.F. Uridine diphosphate glucose: the coenzyme of the galactose-glucose phosphate isomerization. Nature 1950;165:191-192. doi:10.1038/165191a0
- ^ Coelho A.I., Berry G.T., Rubio-Gozalbo M.E. Galactose metabolism and health. Curr Opin Clin Nutr Metab Care 2015;18(4):422-427. doi:10.1097/MCO.0000000000000189
- ^ a b c d Coelho A.I., Rubio-Gozalbo M.E., Vicente J.B., Rivera I. Sweet and sour: an update on classic galactosemia. J Inherit Metab Dis 2017;40(3):325-342. doi:10.1007/s10545-017-0029-3
- ^ a b c d e Conte F., van Buuringen N., Voermans N.C., Lefeber D.J. Galactose in human metabolism, glycosylation and congenital metabolic diseases: time for a closer look. Biochim Biophys Acta Gen Subj 2021;1865(8):129898. doi:10.1016/j.bbagen.2021.129898
- ^ Dall’Olio F. Glycobiology of aging. Subcell Biochem 2018;90:505-526. doi:10.1007/978-981-13-2835-0_17
- ^ a b c Frey P.A. The Leloir pathway: a mechanistic imperative for three enzymes to change the stereochemical configuration of a single carbon in galactose. FASEB J 1996;10(4):461-70. doi:10.1096/fasebj.10.4.8647345
- ^ Garrett R.H., Grisham C.M. Biochemistry. 4th Edition. Brooks/Cole, Cengage Learning, 2010
- ^ a b c d Holden H.M., Rayment I., Thoden J.B. Structure and function of enzymes of the Leloir pathway for galactose metabolism. J Biol Chem 2003;278(45):43885-43888. doi:10.1074/jbc.R300025200
- ^ Leloir L.F., de Fekete M.A., Cardini C.E. Starch and oligosaccharide synthesis from uridine diphosphate glucose. J Biol Chem 1961;236:636-41. doi:10.1016/S0021-9258(18)64280-2
- ^ Pintor J. Sugars, the crystalline lens and the development of cataracts. Biochem Pharmacol 2012;1:4. doi:10.4172/2167-0501.1000e1190
- ^ Rosenthal M.D., Glew R.H. Medical Biochemistry – Human Metabolism in Health and Disease. John Wiley J. & Sons, Inc., 2009
- ^ a b c Thoden J.B., Timson D.J., Reece R.J., and M. Holden H.M. Molecular structure of human galactose mutarotase. J Biol Chem 2004;279(22):23431-23437. doi:10.1074/jbc.M402347200
- ^ Thoden J.B., Wohlers T.M., Fridovich-Keil J.L., Holden H.M. Human UDP-galactose 4-epimerase. Accommodation of UDP-N-acetylglucosamine within the active site. J Biol Chem 2001;276(18):15131-6. doi:10.1074/jbc.M100220200
- ^ Timson D.J. Type IV galactosemia. Genet Med 2019;21:1283-1285. doi:10.1038/s41436-018-0359-z
- ^ Wada Y., Kikuchi A., Arai-Ichinoi N., Sakamoto O., Takezawa Y., Iwasawa S., Niihori T., Nyuzuki H., Nakajima Y., Ogawa E., Ishige M., Hirai H., Sasai H., Fujiki R., Shirota M., Funayama R., Yamamoto M., Ito T., Ohara O., Nakayama K., Aoki Y., Koshiba S., Fukao T., Kure S. Biallelic GALM pathogenic variants cause a novel type of galactosemia. Genet Med 2019;21(6):1286-1294. doi:10.1038/s41436-018-0340-x