Omega-3 polyunsaturated fatty acids or omega-3 PUFAs or omega-3 fatty acids are unsaturated fatty acids, hence lipids, that have a double bond three carbons from the methyl end of the carbon chain.[3]
For humans, the most important omega-3 PUFAs are:
- Alpha-linolenic acid (ALA) or 18:3n-3, with 18 carbon atoms and 3 double bonds;
- eicosapentaenoic acid (EPA) or 20:5n-3, with 20 carbon atoms and 5 double bonds;
- docosahexaenoic acid (DHA) or 22:6n-3, that, with 22 carbon atoms and 6 double bonds, is the most complex.
EPA and DHA are termed long-chain polyunsaturated fatty acids (LC-PUFAs).
Mammals cannot synthesize linoleic acid or LA and alpha-linolenic acid, the precursors to omega-6 polyunsaturated fatty acids and omega-3 PUFAs, respectively, due to the lack of two desaturases: delta-12 desaturase (EC 1.14.19.6) and delta-15 desaturase or omega-3 desaturase or fatty acid desaturase 3 (EC 1-14.19.13).[9] Such desaturases insert double bonds at positions 6 and 3 from the methyl end of the molecule, respectively. Linoleic acid and alpha-linolenic acid are therefore essential fatty acids.[34]
Humans and many other animals can produce, from dietary ALA, all the other omega-3 polyunsaturated fatty acids. Then, such omega-3 PUFAs become essential in the absence of dietary ALA, and for this reason they are termed conditionally essential fatty acids.[9]
EPA and DHA are important structural components of cell membranes, where they are mainly found, especially in muscle and nerve tissues.[35] Conversely, many other fatty acids are stored mainly in adipose tissue triglycerides.
DHA is the main component of cell membrane phospholipids of neural tissues of vertebrates, including photoreceptor of the retina, where it performs important functions.[22][26][29][32] In addition to their structural functions, omega-3 PUFAs are substrates for the production of bioactive lipid mediators with anti-inflammatory action, such as eicosanoids, maresins, resolvins, and protectins.[10][21]
Omega-3 polyunsaturated fatty acids are essential in neurological development of the fetus, and their intake during pregnancy is especially important in the third trimester of pregnancy, when significant brain growth occurs.[8] In the course of life their intake has been associated with a reduction in the risk of developing many chronic diseases, particularly cardiovascular diseases.[1][27][33]
The major dietary sources for humans are fishery products, especially those obtained from cold waters.[3][9][36]
Contents
- Biosynthesis
- Do other animals need EPA and DHA?
- Benefits for humans
- Omega-3/Omega-6 ratio
- Effects at the molecular level of EPA and DHA
- Major sources of EPA and DHA for humans
- Omega-3 and culinary treatments
- References
Biosynthesis
Alpha-linolenic acid is produced from linoleic acid, an omega-6 PUFAs, in the plastids of phytoplankton and vascular terrestrial plants, where delta-15 desaturase inserts a double bond between carbon 3 and 4 from the methyl end of LA.[3][9]
In turn, ALA undergoes desaturation reactions, catalyzed by delta-5 desaturase (EC 1.14.19.44) and delta-6 desaturase (EC 1.14.19.3), elongation reactions, catalyzed by elongase 5 (EC 2.3.1.199) and elongase 5 and/or by elongase 2 (EC 2.3.1.199), and a limited beta-oxidation in peroxisomes, to produce DHA.[6][8]
Note that vascular terrestrial plants do not have the ability to synthesize long chain omega-3 PUFA, such as EPA and DHA.[23]
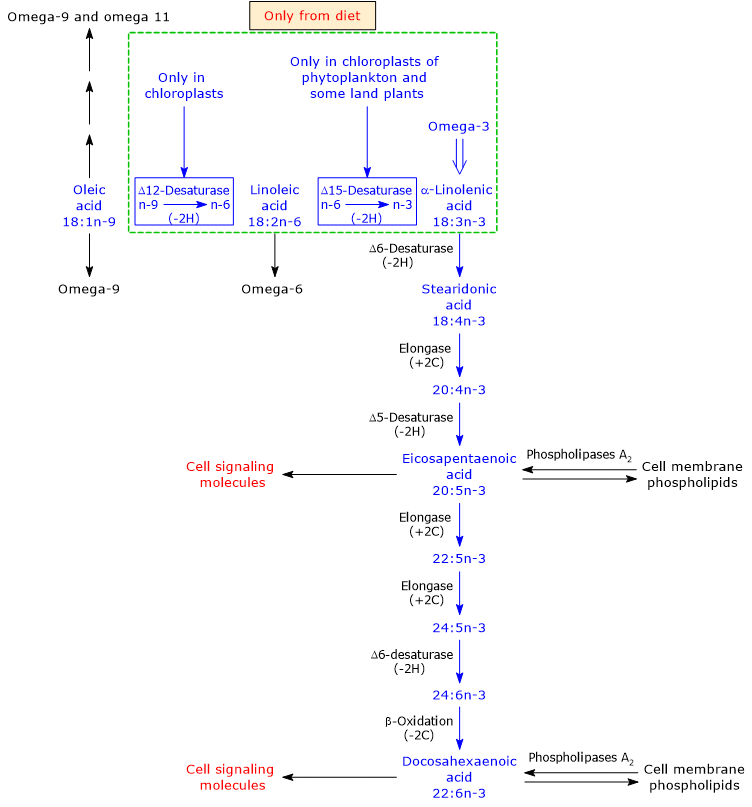
The enzymes that catalyze the conversion of ALA to DHA are shared with the synthetic pathways leading to the synthesis of omega-6, omega-7 and omega-9 PUFAs.[9]
Omega-3 PUFAs appear to be the preferred substrates for delta-5 desaturase and delta-6 desaturase. However, because in many Western diets there is a high intake of linoleic acid relative to alpha-linolenic acid intake, the omega-6 pathway would be preferred over the other pathways.[38] This could be one of the explanations for the low conversion rate of alpha-linolenic acid into the other omega-3 PUFAs, although the synthesis of arachidonic acid (ARA) from linoleic acid seems to be very low, too.
Note that both the omega-3 and omega-6 families inhibit the synthesis of omega-9 polyunsaturated fatty acids.
Omega-3 biosynthesis in humans
Humans, like many other animals, can convert alpha-linolenic acid to docosahexaenoic acid, a metabolic pathway found mainly in the liver and cerebral microcirculation of the hematoencephalic barrier, but also in the cerebral endothelium and astrocytes.[18] It is common opinion that humans, like other terrestrial animals, have a limited capacity to synthesize LC-PUFAs, and therefore need an adequate intake of EPA and DHA from food.[7]
It has been shown that the yield of the synthesis decreases along the pathway: the rate of conversion of alpha-linolenic acid to eicosapentaenoic acid is low, and the limiting factor seems to be the activity of delta-6 desaturase, and the rate of conversion to docosahexaenoic acid is extremely low.[7] However recent studies have demonstrated the existence of a marked polymorphism in the fatty acid desaturase (FADS) gene cluster, especially for the contiguous genes FADS1 and FADS2 coding for delta-5 desaturase and delta-6 desaturase, respectively, which are present on chromosome 11q12.2.[6]
By analyzing genome-wide sequencing data from Bronze Age individuals and present-day Europeans, a comprehensive overview was obtained of the changes in allele frequency of FADS genes.[25]
- In European populations, the transition from a hunter-gatherer society to an agricultural society would have resulted in an increase in the intake of linoleic acid and alpha-linolenic acid, and a reduction in the intake of EPA and ARA. Natural selection would then have favored the haplotype associated with the increase in the expression of FADS1 and the decrease in the expression of FADS2.[6]
- This pattern is opposite to that found in the Greenlander Inuit, where it is hypothesized that natural selection would have favored alleles associated with a decrease in the rate of conversion of linoleic acid and alpha-linolenic acid into LC-PUFAs, in order to compensate for their relatively high dietary intake in such population.[13]
Do other animals need EPA and DHA?
Organisms lacking delta-15 desaturase cannot synthesize alpha-linolenic acid and hence the other omega-3 PUFAs, and, if needed, must obtain it from dietary sources. However, many animals do not need to get EPA and DHA from diet.
Terrestrial herbivorous vertebrates satisfy their need for long chain omega-3 polyunsaturated fatty acids by synthesizing them from alpha-linolenic acid obtained from the green parts of plants.[19]
And there are animals that do not need EPA and DHA, and practically do not have them. These include terrestrial insects, that have very low levels of EPA. In such animals, EPA is synthesized from dietary alpha-linolenic acid and used for eicosanoid production.[16]
Conversely, aquatic insects have high levels of EPA, whereas DHA is practically absent.[31]
Some classes of phytoplankton, such as Cryptophyceae and Dinophyceae, are very rich in EPA and DHA, whereas Bacillariophyceae or diatoms are very rich in EPA. In general, microalgae are the primary producers of EPA and DHA, and then, aquatic ecosystems are the main source of omega-3 LC-PUFAs in the biosphere.[2][28] EPA and DHA are then transferred from these microalgae along the food chain, from invertebrates to fish, and from fish to terrestrial animals, including humans. So, from microalgae to humans.[16]
Benefits for humans
Omega-3 polyunsaturated fatty acids are essential components of a healthy and balanced diet.[36] They are needed throughout development, starting from fetal life, and are associated with health improvements and reduced risk of disease.[8][26]
Indeed, many epidemiological studies have associated high intake of EPA and DHA with a lower cardiovascular mortality, especially for cardiac diseases, than predicted, probably due to the improvements in many risk factors such as plasma levels of triglycerides, HDL-cholesterol, C-reactive protein, blood pressure, both systolic and diastolic, and heart rate.[1][27]
EPA and DHA have also been shown to be useful in the treatment of diseases such as rheumatoid arthritis, and could be useful in the treatment of other inflammatory conditions such as asthma, psoriasis or inflammatory bowel disease, due to their ability to modulate many aspects of the inflammatory processes.[33]
Conversely, LC-PUFAs seem to have little or no effects on measures of glucose metabolism, such as insulin, insulin resistance, fasting glucose, and glycated haemoglobin, or on type 2 diabetes.[5]
Omega-3/Omega-6 ratio
Epidemiological studies suggest that the consumption of a diet with a low omega-3/omega-6 ratio has had a negative impact on human health, contributing to the development, together with other risk factors such as sedentary life and smoking, of the main classes of diseases.[8]
Indeed, a lower incidence of cancer, autoimmunity and coronary heart disease has been observed in populations whose diet has a high omega-3/omega-6 ratio, such as Eskimos and Japanese, populations with a high fish consumption.[20][37]
Despite these evidences, Western diet has become rich in saturated fatty acids and omega-6 polyunsaturated fatty acids, and poor in omega-3 polyunsaturated fatty acids, with an omega-3/omega-6 ratio between 1:10 and 1:20, then, far from the recommended ratio of 1:5.[33]
The low value of the omega-3/omega-6 ratio is due to several factors, some of which are listed below.
- Although wild plant foods are generally high in omega-3 PUFAs, crops high in omega-6 PUFAs have been much more successful in industrial agriculture than those high in omega-3 PUFAs.[3]
- Low consumption of fishery products and fish oils.[33]
- The high consumption of animals raised on corn-based feed, such as chickens, cattle, and pigs. Added to this is the fact that the omega-3 PUFA content of some farmed fish species is lower than that of their wild counterparts.[9]
- The high consumption of oils rich in omega-6 PUFAs and poor in omega-3 PUFAs, such as safflower, sunflower, soybeans and corn oils.[4]
Note: there is no evidence that the omega-3/omega-6 ratio is important for prevention and treatment of type 2
diabetes mellitus.[5]
Effects at the molecular level of EPA and DHA
In recent years, the molecular mechanisms underlying the functional effects attributed to omega-3 polyunsaturated fatty acids, especially to EPA and DHA, are being clarified, and most of these require their incorporation into membrane phospholipids.[23]
Omega-3 PUFAs are structural components of cell membranes where they play an essential role in regulating fluidity.[35] Due to this effect, omega-3, especially EPA and DHA, can modulate cellular responses that depend upon membrane protein functions. This is particularly important in the eye, where DHA allows for optimal activity of rhodopsin, a photoreceptor protein.[29] The effect on membrane fluidity is essential for animals living in cold water, as EPA and DHA also have an antifreeze function.
EPA and DHA can modify the formation of lipid raft, microdomains with a specific lipid composition that act as platforms for receptor activities and the initiation of intracellular signaling pathways. By modifying lipid raft formation, they affect intracellular signaling pathways in different cell types, such as neurons, immune system cells, and cancer cells. In this way, EPA and DHA can modulate the activation of transcription factors, such as NF-κB, PPARs and SREBPs, and so the corresponding gene expression patterns. This is central to their role in controlling adipocyte differentiation, the metabolism of fatty acids and triacylglycerols, and inflammation.[8]
ARA, EPA, and DHA are substrates for the synthesis of bioactive lipid mediators, such as eicosanoids, that are involved in the regulation of inflammation, immunity, platelet aggregation, renal function, and smooth muscle contraction.[21]
Eicosanoids produced from arachidonic acid, that is the major substrate for their synthesis, have important physiological roles, but an excessive production has been associated with numerous disease processes.[8] The increase in EPA and DHA content in membrane phospholipids is paralleled by a reduction in ARA content and associated with a decreased production of lipid mediators form ARA and an increased production of lipid mediators from the two omega-3. Moreover, among the molecules derived from EPA and DHA, there are eicosanoids analogous to those produced from ARA, but with lower activity, resolvins, and, from DHA, protectins and maresins. These molecules appear to be responsible for many of the immune-modulating and anti-inflammatory actions attributed to the omega-3 polyunsaturated fatty acids EPA and DHA.[10]
EPA and DHA can also play a role in the non-esterified form, acting directly through receptors coupled to G proteins, modulating their activity.[30]
Finally, they can reduce the intestinal absorption of omega-6 PUFAs, and, at the enzymatic level, competitively inhibit cyclooxygenase-1 or COX-1 (EC 1.14.99.1) and lipoxygenases, and compete with omega-6 PUFAs for acyltransferases.[3]
Major sources of EPA and DHA for humans
In general, fish and aquatic invertebrates, such as molluscs and crustaceans, are the major sources of EPA and DHA for humans.[3][9][36] These animals can get EPA and DHA from food, namely, from phytoplankton, or synthesize them from alpha-linolenic acid.[16] Moreover, DHA is present in high concentrations in many fish oils, too, especially those from coldwater fish. However, it should be underscored that such oils are also high in saturated fatty acids.[24]
For those who do not eat fishery products, good sources of omega-3 LC-PUFAs are the liver of terrestrial animals and several birds of the order Passeriformes.[14][15]
Regarding the recommended intake of omega-3 polyunsaturated fatty acids, it is not yet clear what it is. The following table shows the values suggested by Food and Agriculture Organization (FAO) of the United Nations and the European Food Safety Authority (EFSA).[11][12]
Organization | Subgroup | Recommendation |
---|---|---|
FAO | Adult males and non-pregnant or non-lactating adult females | Minimum of 250 mg EPA + DHA daily |
Pregnant or lactating females | Minimum of 300 mg EPA + DHA daily of which at least 200 mg should be DHA | |
Children aged 2-4 years | 100-150 mg EPA + DHA daily | |
Children aged 4-6 years | 150-200 mg EPA + DHA daily | |
Children aged 6-10 years | 200-250 mg EPA + DHA daily | |
EFSA | Adult males and non-pregnant adult females | Adequate intake is 250 mg EPA + DHA daily |
Pregnant females | An additional 100-200 mg DHA daily | |
Infants and children aged 6 months to 2 years | 100 mg DHA daily | |
Children aged 2-18 years | ‘‘Consistent with adults’’ |
Omega-3 and culinary treatments
As omega-3 polyunsaturated fatty acids are particularly susceptible to oxidation due to heating, cooking and other culinary treatments could reduce their content.[3] However, this is only partially true. In food, EPA and DHA are not in free form but mainly esterified into membrane phospholipids and, in such form, are much less susceptible to oxidation.[9]
Considering the content of EPA and DHA, to express it as a percentage of the total fatty acids instead of as absolute content, namely, mg/g wet weight, leads to erroneous conclusions. For example, a fatty fish like salmon has a high EPA + DHA content, ~8 mg/g wet weight, and expressed as a percentage of the total fatty acids ~20 percent; conversely Atlantic code has a low EPA + DHA content, ~3 mg/g of wet weight, but, if expressed as a percentage of the total fatty acids ~40 percent. Atlantic code has a high percentage of EPA + DHA because is a lean fish, whereas in fatty fish EPA + DHA content is diluted by the high fatty acid content of the adipose tissue of the animal.[17]
And when EPA + DHA content is expressed in mg/g of product, no decrease in LC-PUFAs content is observed following most common culinary treatments.[16]
References
- ^ a b AbuMweis S., Jew S., Tayyem R. Agraib L. Eicosapentaenoic acid and docosahexaenoic acid containing supplements modulate risk factors for cardiovascular disease: a meta-analysis of randomised placebo-control human clinical. J Hum Nutr Diet 2018;31(1):67-84. doi:10.1111/jhn.12493
- ^ Ahlgren G., Lundstedt L., Brett M., Forsberg C. Lipid composition and food quality of some freshwater phytoplankton for cladoceran zooplankters. J Plankton Res 1990;12(4):809-18. doi:10.1093/plankt/12.4.809
- ^ a b c d e f g Akoh C.C. and Min D.B. Food lipids: chemistry, nutrition, and biotechnology. 3th ed. 2008
- ^ Blasbalg T.L., Hibbeln J.R., Ramsden C.E., Majchrzak S..F, Rawlings R.R. Changes in consumption of omega-3 and omega-6 fatty acids in the United States during the 20th century. Am J Clin Nutr 2011;93(5):950-62. doi:10.3945/ajcn.110.006643
- ^ a b Brown T.J., Brainard J., Song F., Wang X., Abdelhamid A., Hooper L. Omega-3, omega-6, and total dietary polyunsaturated fat for prevention and treatment of type 2 diabetes mellitus: systematic review and meta-analysis of randomised controlled trials. BMJ 2019;366:l4697. doi:10.1136/bmj.l4697
- ^ a b c Buckley M.T., Racimo F., Allentoft M.E., et al. Selection in Europeans on fatty acid desaturases associated with dietary changes. Mol Biol Evol 2017;34(6):1307-1318. doi:10.1093/molbev/msx103
- ^ a b Burdge G.C., Wootton S.A. Conversion of α-linolenic acid to eicosapentaenoic, docosapentaenoic and docosahexaenoic acids in young women. Br J Nutr 2002;88(4):411-420. doi:10.1079/BJN2002689
- ^ a b c d e f Calder P.C. Very long-chain n-3 fatty acids and human health: fact, fiction and the future. Proc Nutr Soc 2018 77(1):52-72. doi:10.1017/S0029665117003950
- ^ a b c d e f g h Chow Ching K. Fatty acids in foods and their health implication. 3th ed. 2008
- ^ a b Duvall M.G., Levy B.D. DHA- and EPA-derived resolvins, protectins, and maresins in airway inflammation. Eur J Pharmacol 2016;785:144-155. doi:10.1016/j.ejphar.2015.11.001
- ^ EFSA Panel on Dietetic Products, Nutrition, and Allergies (NDA). Scientific opinion on dietary reference values for fats, including saturated fatty acids, polyunsaturated fatty acids, monounsaturated fatty acids, trans fatty acids, and cholesterol. 2010. doi:10.2903/j.efsa.2010.1461
- ^ FAO. Global recommendations for EPA and DHA intake (As of 30 June 2014)
- ^ Fumagalli M., Moltke I., Grarup N., Racimo F., Bjerregaard P., Jørgensen M.E., Korneliussen T.S., Gerbault P., Skotte L., Linneberg A., Christensen C., Brandslund I., Jørgensen T., Huerta-Sánchez E., Schmidt E.B., Pedersen O., Hansen T., Albrechtsen A., Nielsen R.. Greenlandic Inuit show genetic signatures of diet and climate adaptation. Science 2015;349(6254):1343-7. doi:10.1126/science.aab2319
- ^ Gladyshev M.I., Makhutova O.N., Gubanenko G.A., Rechkina E.A., Kalachova G.S., Sushchik N.N. Livers of terrestrial production animals as a source of long-chain polyunsaturated fatty acids for humans: an alternative to fish? Eur J Lipid Sci Technol 2015;117:1417-1421. doi:10.1002/ejlt.201400449
- ^ Gladyshev M.I., Popova O.N., Makhutova O.N., Zinchenko T.D., Golovatyuk L.V., Yurchenko Y.A., Kalachova G.S., Krylov A.V., Sushchik N.N. Comparison of fatty acid compositions in birds feeding in aquatic and terrestrial ecosystems. Contemp Probl Ecol 2016;9:503-513. doi:10.1134/S1995425516040065
- ^ a b c d Gladyshev M.I. and Sushchik N.N. Long-chain omega-3 polyunsaturated fatty acids in natural ecosystems and the human diet: assumptions and challenges. Biomolecules 2019;9(9):485. doi:10.3390/biom9090485
- ^ Gladyshev M.I., Sushchik N.N., Tolomeev A.P., Dgebuadze Y.Y. Meta-analysis of factors associated with omega-3 fatty acid contents of wild fish. Rev Fish Biol Fish 2018;28,:277-299. doi:10.1007/s11160-017-9511-0
- ^ Kim H-Y. Novel metabolism of docosahexaenoic acid in neural cells. J Biol Chem 2007;282(26):18661-18665. doi:10.1074/jbc.R700015200
- ^ Kouba M., Mourot J. A review of nutritional effects on fat composition of animal products with special emphasis on n-3 polyunsaturated fatty acids. Biochimie 2011;93(1):13-7. doi:10.1016/j.biochi.2010.02.027
- ^ Kromann N., Green A. Epidemiological studies in the Upernavik district, Greenland. Incidence of some chronic diseases 1950-1974. Acta Med Scand 1980;208(5):401-6. doi:10.1111/j.0954-6820.1980.tb01221.x
- ^ a b Kuda O. Bioactive metabolites of docosahexaenoic acid. Biochimie 2017;136:12-20. doi:10.1016/j.biochi.2017.01.002
- ^ Lauritzen L., Hansen H.S., Jørgensen M.H., Michaelsen K.F. The essentiality of long chain n-3 fatty acids in relation to development and function of the brain and retina. Prog Lipid Res 2001;40(1-2):1-94. doi:10.1016/s0163-7827(00)00017-5
- ^ a b Lee J.M., Lee H., Kang S. and Park W.J. Fatty acid desaturases, polyunsaturated fatty acid regulation, and biotechnological advances. Nutrients 2016;8(1):23. doi:10.3390/nu8010023
- ^ Mason R.P., Sherratt S.C.R. Omega-3 fatty acid fish oil dietary supplements contain saturated fats and oxidized lipids that may interfere with their intended biological benefits. Biochem Biophys Res Commun 2017;483(1):425-429. doi:10.1016/j.bbrc.2016.12.127
- ^ Mathieson I., Lazaridis I., Rohland N., Mallick S., Patterson N., Roodenberg S.A., Harney E., Stewardson K., Fernandes D., Novak M., et al. Genome-wide patterns of selection in 230 ancient Eurasians. Nature 2015;528:499-503. doi:10.1038/nature16152
- ^ a b McCann J.C., Ames B.N. Is docosahexaenoic acid, an n-3 long-chain polyunsaturated fatty acid, required for development of normal brain function? An overview of evidence from cognitive and behavioral tests in humans and animals. Am J Clin Nutr 2005;82(2):281-95. doi:10.1093/ajcn.82.2.281
- ^ a b Mensink R.P., Zock P.L., Kester A.D., Katan M.B. Effects of dietary fatty acids and carbohydrates on the ratio of serum total to HDL cholesterol and on serum lipids and apolipoproteins: a meta-analysis of 60 controlled trials. Am J Clin Nutr 2003;77(5):1146-55. doi:10.1093/ajcn/77.5.1146
- ^ Muller-Navarra D.C. Biochemical versus mineral limitation in Daphnia. Limnol Oceanogr 1995;40:1209-1214. doi:10.4319/lo.1995.40.7.1209
- ^ a b Niu S.L., Mitchell D.C., Lim S.Y., Wen Z.M., Kim H.Y., Salem N. Jr, Litman B.J. Reduced G protein-coupled signaling efficiency in retinal rod outer segments in response to n-3 fatty acid deficiency. J Biol Chem 2004;279(30):31098-104. doi:10.1074/jbc.M404376200
- ^ Oh D.Y., Talukdar S., Bae E.J., Imamura T., Morinaga H., Fan WQ, Li P., Lu W.J., Watkins S.M., Olefsky J.M. GPR120 is an omega-3 fatty acid receptor mediating potent anti-inflammatory and insulin-sensitizing effects. Cell 2010 142(5):687-698. doi:10.1016/j.cell.2010.07.041
- ^ Popova O.N., Haritonov A.Y., Sushchik N.N., Makhutova O.N., Kalachova G.S., Kolmakova A.A., Gladyshev M.I. Export of aquatic productivity, including highly unsaturated fatty acids, to terrestrial ecosystems via Odonata. Sci Total Environ 2017;581-582:40-48. doi:10.1016/j.scitotenv.2017.01.017
- ^ Salem N. Jr, Litman B., Kim H.Y., Gawrisch K. Mechanisms of action of docosahexaenoic acid in the nervous system. Lipids 2001;36(9):945-59. doi:10.1007/s11745-001-0805-6
- ^ a b c d Simopoulos A.P. The importance of the omega-6/omega-3 fatty acid ratio in cardiovascular disease and other chronic diseases. Exp Biol Med 2008;233(6):6746-88. doi:10.1016/S0753-3322(02)00253-6
- ^ Smith W., Mukhopadhyay R. Essential fatty acids: the work of George and Mildred Burr. J Biol Chem 2012;287(42):35439-35441. doi:10.1074/jbc.O112.000005
- ^ a b Stubbs C.D., Smith A.D. The modification of mammalian membrane polyunsaturated fatty acid composition in relation to membrane fluidity and function. Biochim Biophys Acta 1984;779(1):89-137. doi:10.1016/0304-4157(84)90005-4
- ^ a b c Tocher D.R., Betancor M.B., Sprague M., Olsen R.E., Napier J.A. Omega-3 long-chain polyunsaturated fatty acids, EPA and DHA: bridging the gap between supply and demand. Nutrients 2019;11(1):89. doi:10.3390/nu11010089
- ^ Yano K., MacLean C.J., Reed D.M., Shimizu Y., Sasaki H., Kodama K., Kato H., Kagan A. A comparison of the 12-year mortality and predictive factors of coronary heart disease among Japanese men in Japan and Hawaii. Am J Epidemiol 1988;127(3):476-87. doi:10.1093/oxfordjournals.aje.a114824
- ^ Williams C.M., Burdge G. Long-chain n-3 PUFA: plant v. marine sources. Proc Nutr Soc 2006;65(1):42-50. doi:10.1079/pns2005473